Research highlight
Fiber-based sensor sees single nanoparticles
There is a need for compact, cheap, and accurate devices to detect nanoparticles like pathogens, biomarkers, and ultrafine particles polluting the air. In an article in the journal Optica, PhD researcher Arthur Hendriks from the Photonics and Semiconductor Nanophysics group demonstrated a nanophotonic fiber-tip sensor that is able to detect single particles down to 50 nanometers in size, in real-time.
The research started with the work of his colleague Luca Picelli, who developed a simple and reproducible method to transfer semiconductor membranes onto the facet of a cleaved optical fiber, Arthur Hendriks starts telling the tale of his technological breakthrough. ‘In my PhD project, I explored the possibilities of this transfer technique to fabricate sensors, for example by attaching optomechanical devices or photonic crystal cavities onto a glass fiber tip.’ Fiber-optic sensors have multiple advantages over other sensing techniques: the fibers are cheap, they enable measurements at a distance, and they are insensitive to electromagnetic interference. There have been many demonstrations of fiber sensors already. However, the detection of ultrasmall objects such as nanoparticles has remained elusive, since they are way smaller than the diffraction limit and therefore difficult to detect using light.
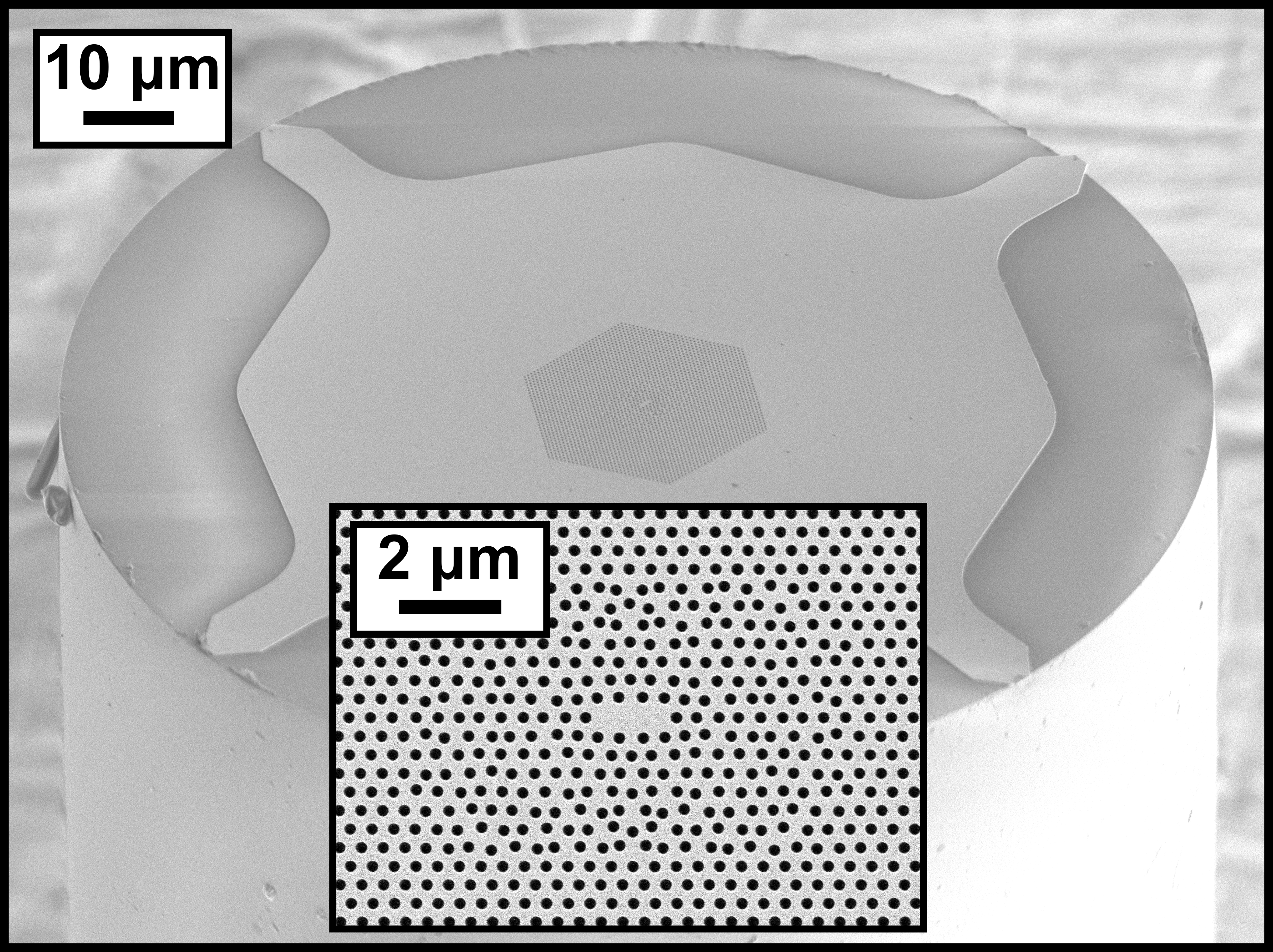
The photonic crystal he used consists of an indium phosphide membrane containing periodically located air holes that define a bandgap – a band of frequencies for which no propagation is possible.
Trapping light
In an attempt to make a sensitive particle sensor, Hendriks integrated a so-called photonic crystal cavity on top of a fiber. The photonic crystal he used consists of an indium phosphide membrane containing periodically located air holes that define a bandgap – a band of frequencies for which no propagation is possible. By strategically leaving some of the holes out in the center of the crystal, a photonic nanocavity is created. This optical cavity can trap light of a certain wavelength. When a tiny particle comes close to the cavity, the resonant wavelength of the cavity will shift to a higher value. This tiny wavelength shift can be measured, by sweeping a laser over the resonant wavelength of the crystal and detecting the reflected light through the glass fiber.
In order to make a sensitive sensor based on this idea, the challenge is to trap light as tightly as possible in space and as long as possible in time. In their experiment, Hendriks and his colleagues killed multiple birds with one stone: by using the membrane transfer method previously developed in the group, they managed to effectively couple the light from the fiber to the cavity and back. This enabled a sensitive measurement of the cavity wavelength through the fiber. What’s more, they designed an optical cavity that had the right combination of light confinement properties to do the trick. ‘That is the part I personally liked best about this research,’ Hendriks says. ‘By using a technique developed at Stanford University to optimize the design of the photonic crystal, we ended up with a design that consists of equally sized holes with a very specific spatial distribution close to the cavity. Our first simulations of the crystal’s expected performance seemed too good to be true. But when we fabricated and tested it, our experiment turned out to match our simulations very well! That was a satisfying result since any minor defect that arises during fabrication has a significant effect on the accuracy of the wavelength measurement and the amount of light that can be detected.’
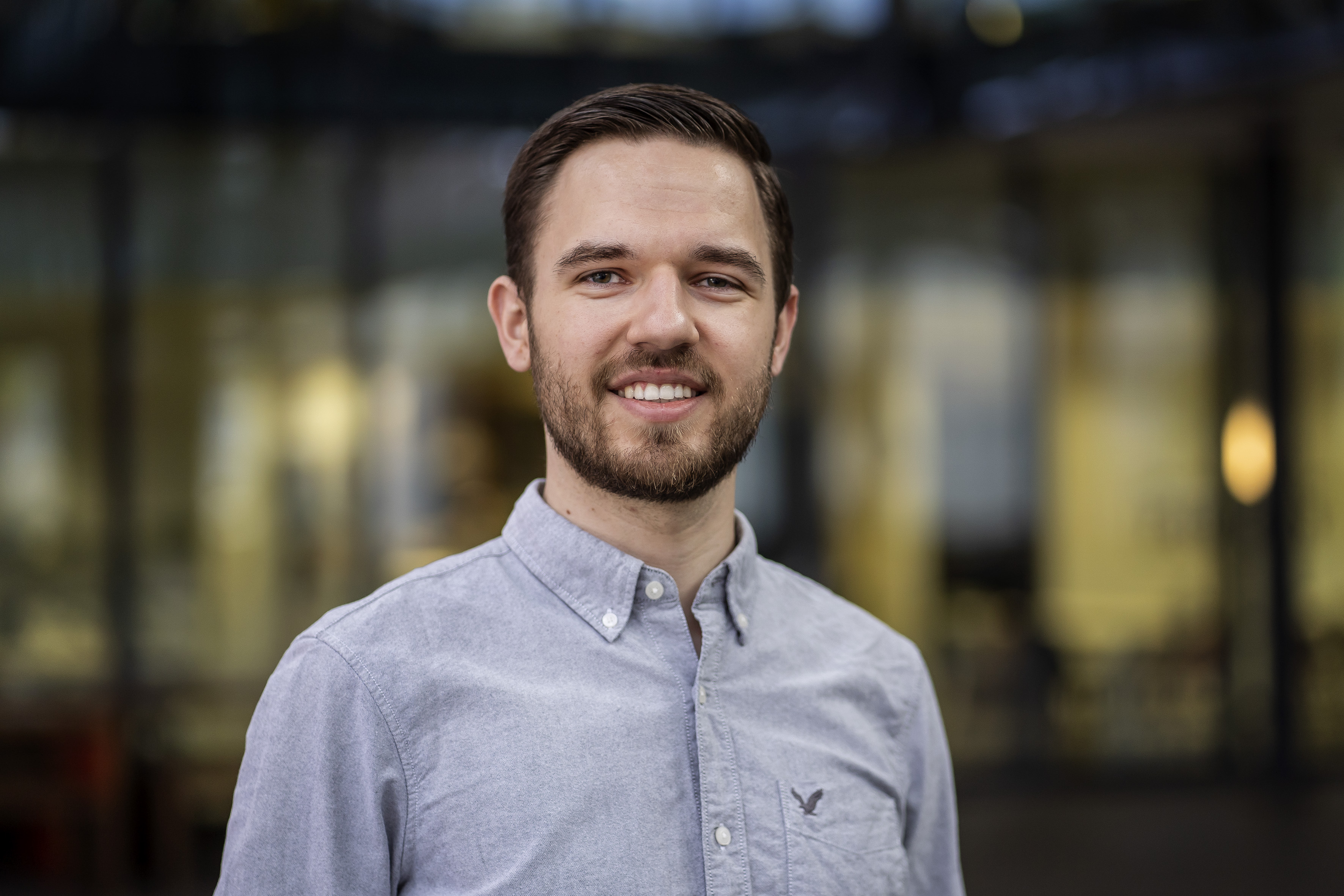
Perhaps by leaving out only one, or even adding an extra hole, we could confine the electric field even further. That way, we could probably detect even smaller particles, perhaps even down to single biomolecules like antibodies.
Arthur Hendriks | PhD Candidate
View on single particles
The researchers demonstrated that their system is able to observe discrete redshifts in the wavelength of the cavity whenever a nanoparticle is close to the optical cavity. In their experiments, Hendriks and his colleagues used polystyrene spheres suspended in water. With their fiber-tip sensor, they could accurately and reproducibly detect particles measuring just 50 nanometers across, which corresponds for example with the size of ultrafine particles that can cause health issues when airborne. To assess the added value of this measurement technique in practice, it is going to be investigated in the European project LEARN to monitor the air quality in closed spaces such as schools.
In the meanwhile, the group is further developing this technique, Hendriks says. ‘In this paper, we have shown that this first design already works rather well, but of course, there is still ample room for improvement.’ For example, it could be greatly beneficial to not directly stick the photonic crystal membrane onto the fiber tip, but to slightly suspend it above the tip instead. In addition to that, Hendriks sees possibilities to improve the read-out of the reflected light signals. ‘Now, we are using off-the-shelf commercially available systems. I can imagine that we can optimize the readout by designing a tailormade setup instead.’ Changing the fibers to catch more of the reflected light could further improve the measurements. ‘And our choice to leave out three holes to construct the optical cavity is not the only way to do it. Perhaps by leaving out only one, or even adding an extra hole, we could confine the electric field even further. That way, we could probably detect even smaller particles, perhaps even down to single biomolecules like antibodies.’
Harnessing ultrafast heat
Integrating polymers to push photonics
Fiber-based sensor sees single nanoparticles